The Gardener Monk’s Genetic Revolution:
From Mendel’s pea plants to
the breakthroughs of CRISPR
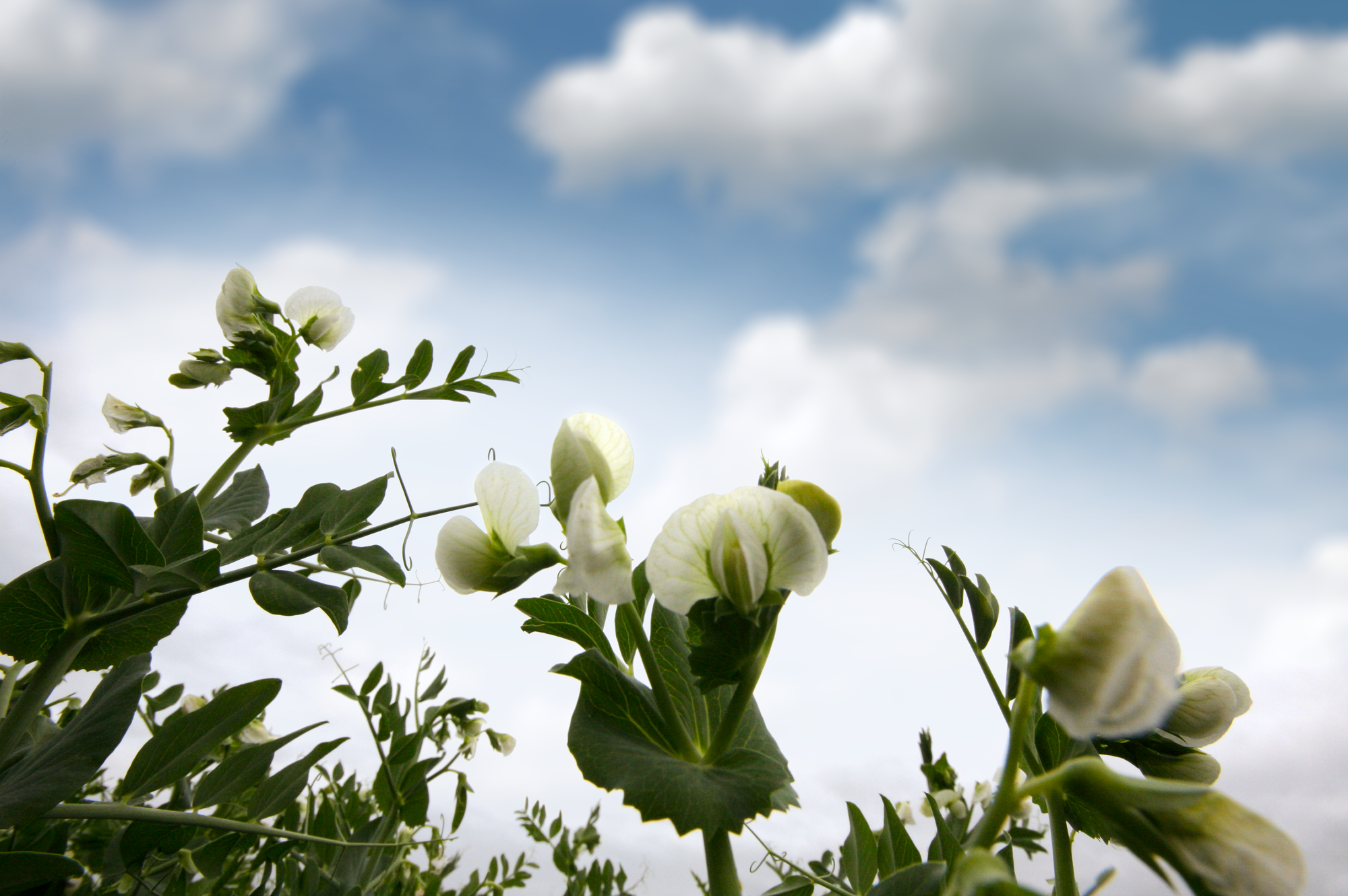
On the chilly evening of February 8, 1865, in what is now the Czech Republic city of Brno, as lamplighters lit the gas streetlights, a shy part-time science teacher made his way to a second-floor classroom to address the monthly meeting of the Natural Science Society.
Previous lecturers had covered topics as diverse as geology, meteorology and mathematics. But the meeting that night would turn out to be far more important. The speaker was Johann Gregor Mendel, who was also a monk at the local monastery, where he spent much of his time in the gardens.
Mendel’s presentation of his paper, 'Experiments on Plant Hybridization', was well received by the local amateur scientists and was even briefly covered in the local German-language newspapers. But it was then largely ignored, being cited by scientists only a handful of times over the next three decades.
And yet, even if the 40 attendees that February evening didn’t realize it, Mendel’s paper was a scientific breakthrough, foundational to the creation of modern genetics. It heralded an agricultural revolution that continues to this day in the way the world’s farmers produce our food.
Gregor Mendel, photo credit: Wikimedia Commons
Gregor Mendel, photo credit: Wikimedia Commons
Mendel's Experiments
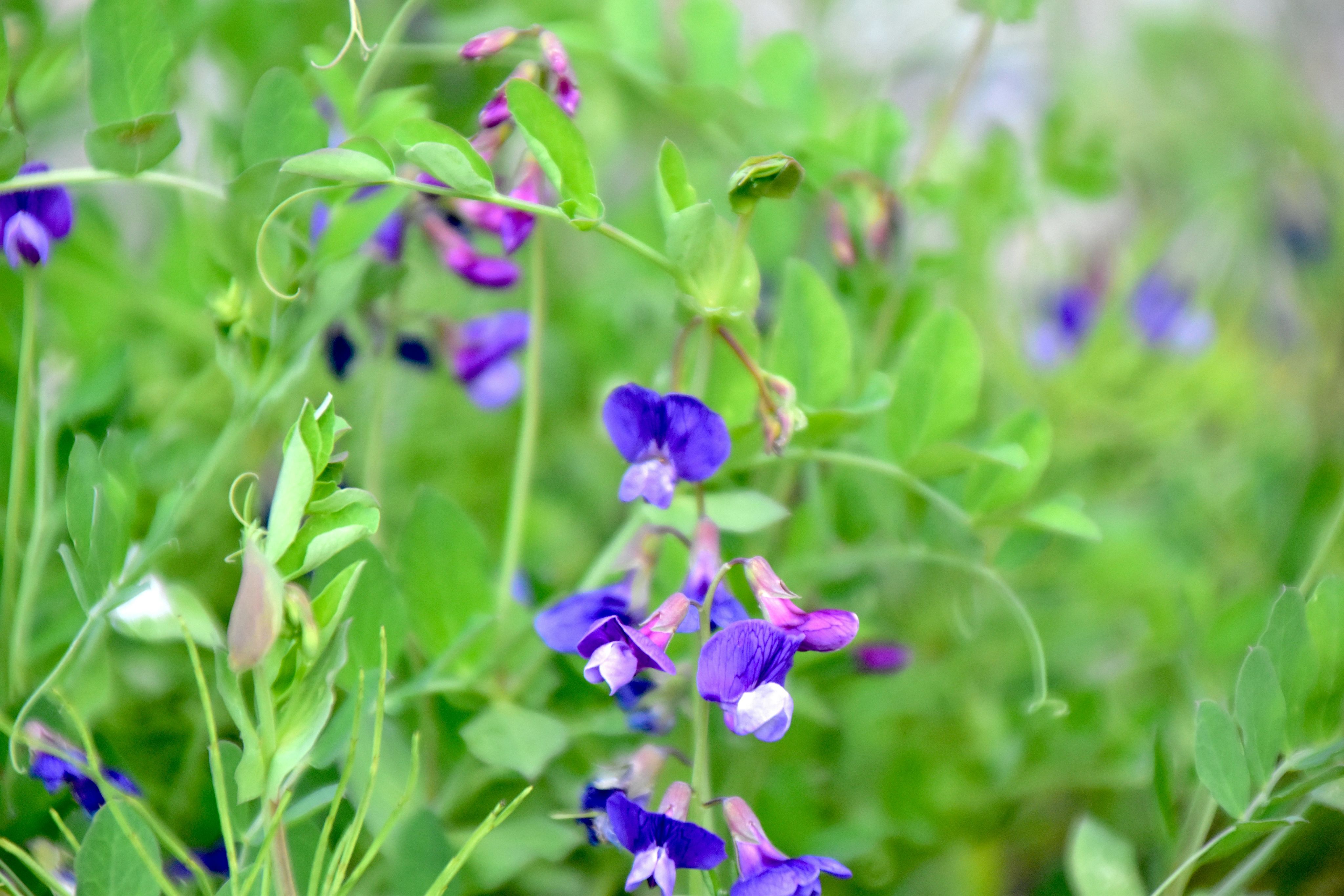
Over the course of eight years, Mendel carried out a series of experiments with pea plants. Armed with a paintbrush, he painstakingly pollinated plants and then observed how certain traits were passed on. His meticulous record keeping and detailed observations allowed Mendel to monitor thousands of pea plants during that period, tracking how seven different traits moved through multiple generations.
These experiments provided scientists with the first insights into dominant and recessive traits. By cross breeding a pure-bred white flower pea plant with a pure-bred purple flower pea plant, the typical 19th-century biologist might expect the next generation’s flowers to be a blend of colors. However, Mendel found that the offspring of that first generation were all purple flowered plants. But when that generation self-fertilized to produce offspring, they yielded — by a ratio of 3:1 — many purple flowers but also some white ones.
From this Mendel developed his theory of hereditary factors – which today we call genes, with the various forms in which those genes are expressed known as alleles. This insight gives rise to one of the Mendelian laws – the law of dominance and uniformity. Mendel claimed that a plant with one dominant allele will display the characteristics of that dominant trait. The implications of Mendel’s experiments would not become clear to the wider world for decades, but his laws derived from observation laid the foundation for finding new traits and improving plants through careful selective breeding.
The Birth of Modern Genetics
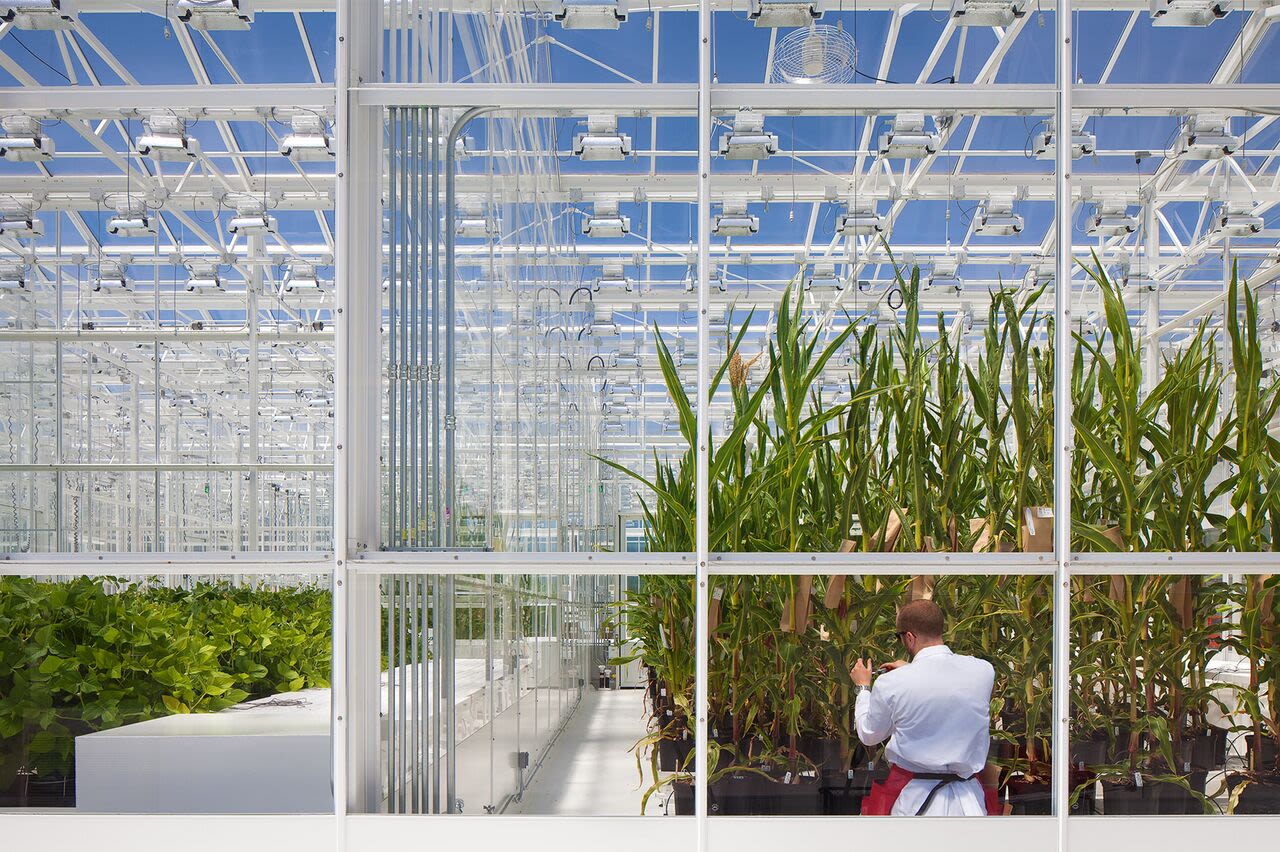
William Bateson, Photo credit: Public domain, via Wikimedia Commons
William Bateson, Photo credit: Public domain, via Wikimedia Commons
Thirty years after Mendel had made his initial observations, various European scientists independently started to reach similar conclusions. Trying to come up with new theories of inheritance, the Dutch scientist Hugo de Vries and the Austrian agronomist Erich von Tschermak each independently realized that Mendel had got there well before them. Finally, the quiet monk’s work was rediscovered, and his results were soon being replicated.
One of the earliest popularizers of Mendel’s ideas was the English scientist William Bateson, the first to suggest the word genetics as a term for the study of inheritance. Bateson formed one of the first unofficial schools of genetics at the University of Cambridge. It was here where some of the first groundbreaking experiments in genetic inheritance and plant breeding took place.
Bateson worked alongside several underappreciated figures in the history of genetic science, such as Muriel Onslow, who worked on the inherited colors of snapdragon flowers, and Florence Durham, who expanded our understanding of Mendelian inheritance through her experiments in mice and the colors of their fur.
Around the same time, scientist Roland Biffen was credited with some of the first studies on the inheritance of disease resistance. In the wake of World War I, there was increasing awareness of the need to fund agricultural research to maintain and secure national food supplies.
Bateson would eventually become the first director of the John Innes Horticultural Institution, a center set up for horticultural experiments and research, with an emphasis on plant genetics. Just 20 years after opening, it launched the first of its many new commercial fruit varieties — three different species of blackberry — resulting from its experiments in plant breeding and inheritance. Centers for plant and genetic research would quickly become common across Europe, the UK and the rest of the world, many of them receiving government funding.
With food shortages becoming a serious issue for much of Europe following World War II, there was increasing awareness of the need for agriculture to change. Yields needed to be higher, and the next decades would see an explosion of production driven by new hybrid varieties, new fertilizers and new technology, which allowed farmers to be more productive than ever. But getting to that point was not an easy process.
The Green Revolution
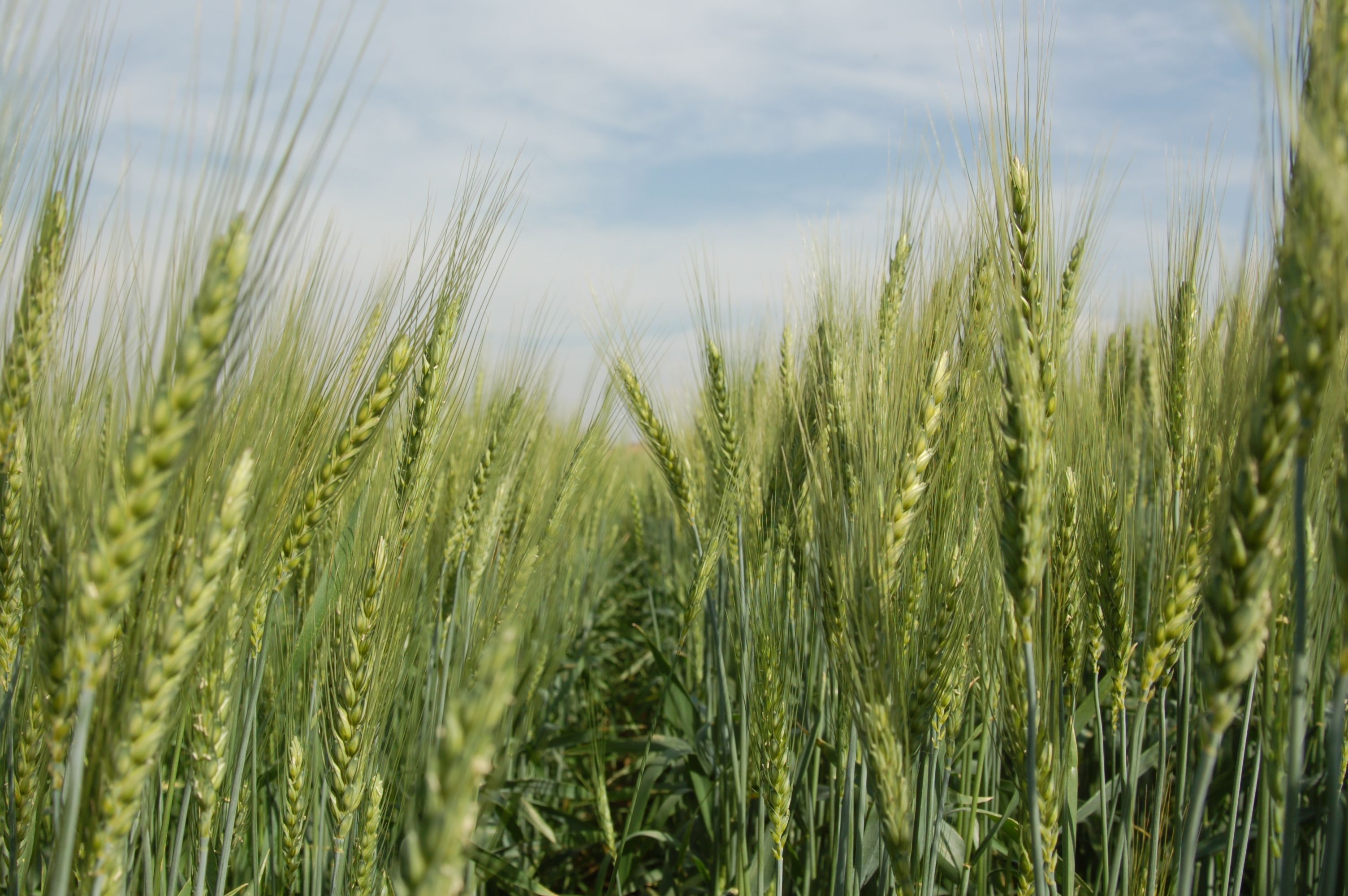
While Europe had struggled to produce enough food in the first half of the 20th century, American agriculture had thrived as US farmers maintained healthy surpluses. Rationing would not end in Britain until the 1950s, and food supplies across the rest of the world were often prone to failures or serious shortages. Research centers around the world became vital for accelerating the discovery, implementation, and adoption of new high-yield crop varieties.
The results were remarkable, as governments worldwide sought to increase yields and guarantee food security.
One of the major turning points came in 1944 when an American agronomist and wheat breeder, Norman Borlaug, was appointed to lead the wheat breeding efforts at the International Maize and Wheat Improvement Center in Mexico. He and his team spent a decade crossbreeding wheat for disease resistance, producing multiple compatible hybrids that all had disease-resistance genes.
After establishing this genetic base, the team encountered a problem: the cultivars produced were long and slender, prone to growth spurts thanks to the nitrogen fertilizer used to supplement the poor soil quality. As a result, the wheat would become top-heavy, collapsing under its own weight.
To solve this, Borlaug and team crossbred their disease-resistant hybrids with a semi-dwarf wheat hybrid producing wheat that was shorter and less prone to collapse, and, thanks to a decade of plant breeding, well suited to the Mexican climate. The two varieties developed were called Pitic 62 and Penjamo 62: they were disease resistant, responsive to chemical inputs and grew to a sustainable height.
The results were nothing short of spectacular, and these new breeds were enthusiastically taken up by farmers. By 1963, around 95% of the wheat crop of Mexico was using these dwarf varieties. That year, the total wheat harvest in the country was six times higher than it had been the year Borlaug arrived in Mexico.From there, Borlaug and team kept refining, producing new varieties, and took his dwarf gene carrying wheat across the world.
In the mid-1960s India was a huge importer of food, mostly sent from the United States. Despite some logistical issues in moving seed from Mexico to India, test plots were soon established, and the harvests were so impressive that both India and Pakistan committed to large imports of wheat seeds with these vital genetic traits. Once again, the results were astounding. Exact figures are difficult to pin down but in 1964 India produced 12 million tons of wheat. By 1968 the figure was 17 million tons. Writing in 2013, 50 years after the first introduction of high yield varieties, the Indian agronomist M.S Swaminathan pointed out that without those high yield varieties the country would have needed to use three times as much land dedicated to growing wheat. In Pakistan, yields grew from four million tons in 1965 to eight million tons by 1970 with both nations benefitting from the impact of Borlaug’s high yielding crops.
Borlaug, along with figures like Swaminathan and India’s Minister for Agriculture Chidambaram Subramaniam, were credited with kicking off a 'green revolution'. The combination of fertilizers, new technology and the crucial breakthrough of high-yield plant breeds has been credited with saving upward of a billion people from starvation. Swaminathan was awarded the first ever World Food Prize (one of the highest agricultural honors) and Borlaug was awarded the 1970 Nobel Peace Prize for his contribution to helping provide more food for so much of the world.
Norman Borlaug, Photo credit: Ben Zinner, USAID, Public domain, via Wikimedia Commons
Norman Borlaug, Photo credit: Ben Zinner, USAID, Public domain, via Wikimedia Commons
Monkombu Sambasivan Swaminathan, Photo credit: Biswarup Ganguly, CC BY 3.0 , via Wikimedia Commons
Monkombu Sambasivan Swaminathan, Photo credit: Biswarup Ganguly, CC BY 3.0 , via Wikimedia Commons
CRISPR and the Future of Plant Breeding
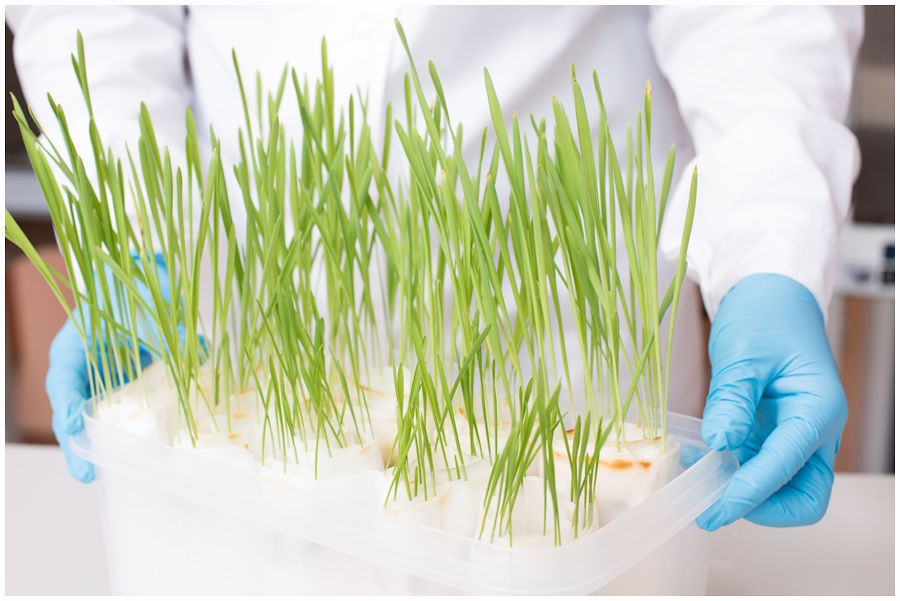
Despite these breakthroughs, the Green Revolution is not something that could, or should, be repeated. An over-reliance on specific varieties, combined with a heavy use of chemicals did achieve success, but the climatic and environmental challenges of the future will demand further, but more sustainable, innovation.
As more secrets are unlocked through the science of genetics – far more than figures like Bateson could have dreamed – researchers have developed new techniques to precisely refine genetic traits. These breakthroughs have the potential to greatly speed up the breeding process and cut the time it takes to move a new trait through breeding trials and on to commercialization.
The 1970s and 1980s saw the development of GMO (genetically modified organism) technology, a huge step beyond the paintbrush experiments of Mendel. GMO technology allowed scientists to introduce a gene from one organism into another, directly modifying the recipient’s DNA. Uses for the world of agriculture were quickly seized upon, with the first herbicide-tolerant crops being released in the 1980s.
Genetic modification can also be used to increase the nutrient value of food. Take Golden rice: this is a rice crop that has been modified with three genes that biosynthesis beta-carotene, which the human body metabolizes as vitamin A. This rice variety is aimed at helping mitigate vitamin A deficiency, which can be a cause of child mortality.
GMO technology has been increasingly politicized and subject to a wide range of regulations across the world. Despite this, scientific advancement has continued, and techniques of genome editing have the potential to be transformative on agricultural production.
The difference between genetic modification and genome editing is important. Genome editing allows for scientists to make small modifications to a plant’s genetics but doesn’t introduce genetic material from another organism.
The most well-known genome editing technique was the Nobel Prize winning development of CRISPR (Clustered Regularly Interspaced Short Palindromic Repeats). CRISPR is essentially a pair of molecular scissors, that allows scientists to cut a target DNA.
At Syngenta, a scientific team led by Tim Kelliher, Head of Technology Development, developed a gene editing technique based on CRISPR technology. The Syngenta technique is called Haploid Induction editing — or Hi-Edit for short. Hi-Edit enables direct genomic modification of commercial crop varieties. The technique is a boon for plant breeding as it has the potential to greatly speed up the process of developing and testing new traits.
Mendel only went through a few generations in his pea plant experiments (and that took eight long years). The contemporary processes of trait selection, breeding and field trials can take 15 to 20 years to bring a new trait to market. With Hi-Edit, this could be reduced by five to 10 years.
Genomic editing could allow for crops that are not as dependent on fertilizers or have genetic resistance to disease, requiring less pesticides. Genomic editing could also lead to crops with higher yields and a longer shelf life, cutting down food waste and boosting the profitability of farmers.
With the global population predicted to hit 10 billion people before the end of the century, society must find ways of boosting food production and do so in a sustainable way. Yet even with the new technology that has emerged — including genome editing and data monitoring of field trials — much of plant breeding remains indebted to the insights and observations made by Gregor Mendel in his monastery garden, more than 150 years ago.